Radioactivity mapping of beach sand by mobile in situ gamma-ray spectrometry
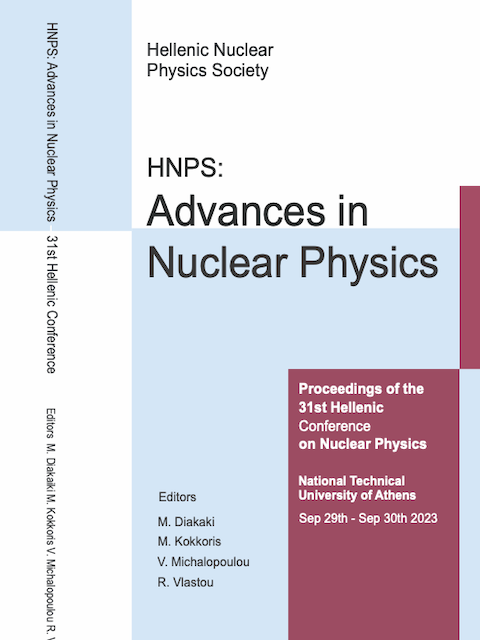
Abstract
A new method for prompt radioactivity mapping of beach sand is under development. It is based on mobile in situ gamma-ray spectrometry exploiting low- and medium-resolution portable scintillator systems. Two case study applications are presented which took place during IAEA’s RER1020 and CRP F22074 projects, aiming to determine the main methodological features, capabilities, and limitations of the new method. In general, spectra of very short acquisition time (20s) are obtained by a mobile unit (man or vehicle) along transects on the beach sand. The statistic of each spectrum is extremely low for individual analysis however, the spatial resolution of each measurement is preserved high (a few meters). The distribution of the total counting rate is used to classify the spectra, according to quartiles, into four classes (high, low, medium-high, and medium-low). For each class, the spectra are combined into one of a total acquisition time high enough for spectrometric analyses. Laboratory HPGe measurements and simulation studies were used to calculate the efficiency of selected photo-peak energies. The first maps obtained by the mobile method are in very good agreement with those obtained by grid sampling and laboratory analyses. Critical aspects under further investigation regard the varied physical parameters of the beach sand (density, water content, porosity) and the complicated detection geometry both of them strongly related to the detection efficiency.
Article Details
- How to Cite
-
Patiris, D., Tsabaris, C., Maramathas, C., Alexakis, S., & Roumelioti, S. K. (2024). Radioactivity mapping of beach sand by mobile in situ gamma-ray spectrometry. HNPS Advances in Nuclear Physics, 30, 116–123. https://doi.org/10.12681/hnpsanp.6269
- Issue
- Vol. 30 (2024): HNPS2023
- Section
- Oral contributions

This work is licensed under a Creative Commons Attribution-NonCommercial-NoDerivatives 4.0 International License.